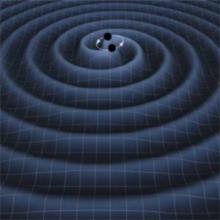
Gravitational waves are ripples in the fabric of the universe, produced when mass accelerates. Because they're hard to produce, and thus conversely hard to obscure, they provide excellent probes into the most energetic phenomena in the universe. Just like different parts of the electromagnetic spectrum have different practical applications, we can use gravitational waves of different scales to probe a wide range of phenomena.
CCRG scientists are involved with several observatories to identify gravitational waves from objects similar in mass to our sun (LIGO and Virgo); from the supermassive black holes in the center of galaxies (PTA, LISA); and from known pairs of stars in our own galaxy (LISA). Each of these experiments identifies gravitational waves from a distinct frequency range, enabling us to measure some of the gravitational-wave radiation from different astrophysical sources. In a few cases, multiple gravitational-wave detectors can be sensitive to the same type of source; in exceptional circumstances, the same source can be identified with multiple gravitational-wave instruments. Most often, however, each instrument provides a unique perspective on some astrophysical phenomena.
Ground-Based Gravitational-Observatories: LIGO
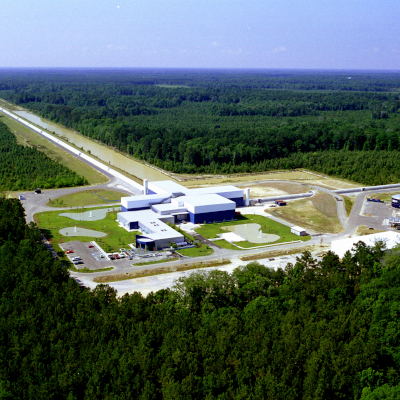
Ground-based gravitational-wave observatories like LIGO and Virgo are particularly sensitive to coalescing objects that derive from massive stars: stellar-origin black holes and neutron stars. RIT researchers work within the LIGO Scientific Collaboration to analyze the data taken by LIGO and other detectors, searching for the signatures of these astrophysical systems.
LIGO is the Laser Interferometer Gravitational Wave Observatory, and the largest project the NSF has ever funded. Built to help prove a key prediction from Einstein’s general relativity,The LIGO scientific collaboration is an international collaboration currently spanning over 1,200 scientists, and 108 institutions and operates two michelson interferometer that measures the phase difference between light travel time when a gravitational wave passes. The interferometers are located in Hanford, Washington, and Livingston, Louisiana. In September 2015, the LIGO scientific collaboration detected the merging of two black holes, and provided a direct test for Einstein's theory, which was awarded the Nobel Prize in 2015. Since then, there have been numerous more binary black hole mergers, and even a binary Neutron star merger. LIGO is sensitive to gravitational wave frequencies from a tens to hundreds of hertz, but future generations of interferometers may manage to reach down to a few hertz or so.
The outgoing gravitational-wave transient signal permits us to deduce each binary's properties: binary masses, spins, and (if matter is present) matter composition. Once current instruments reach design sensitivity, we will identify hundreds of these objects per year; future instruments can observe millions each year. Using the strong constraints on the underlying compact binary mass and spin distribution over cosmic time, these observations will exquisitely constrain how compact binaries form, both their underlying physics and the ingredients involved in producing them like the star formation rate of the universe. CCRG scientists work to characterize the sources responsible for these transients, both individually and as a population. Ground-based gravitational-wave observatories are also sensitive to persistent gravitational-wave sources. One long-expected source is rotating neutron stars in our own galaxy with some small surface asymmetry (a "mountain"). This distortion could be imparted many ways: at birth, imparted during the supernova that formed it; be sustained via large magnetic fields; or imparted via accretion from a companion. Gravitational-wave observations of these continuous sources should enable new insights into dense matter and compact object formation. CCRG scientists work to find these sources in LIGO data.
Space-Based Gravitational Wave Observatories: LISA
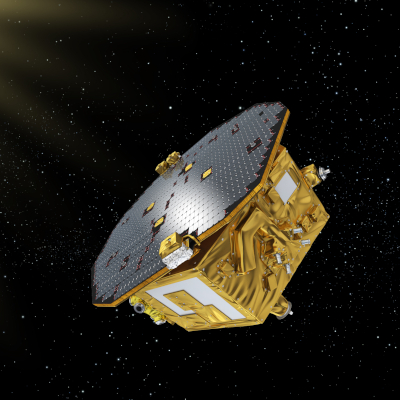
Following the success of LIGO, LISA is the Laser Interferometer Space Antenna, which is a European Space Agency project tasked with detecting gravitational waves. Gravitational waves are ripples in spacetime produced from interactions of massive objects. Today, LIGO is a ground-based detector able to detect gravitational waves from tens to hundreds of hertz, but LISA is the first ever space-based gravitational wave detector whose detectability range is from 0.1 milihertz to roughly 1 hertz. This means that wavelengths are much longer than those detected using LIGO, from systems with much wider orbits and much heavier objects. Planned for early 2030s, LISA’s proposed design is a interferometer shaped in an equilateral triangle consisting of 5 million kilometer long arms. At each point in the triangle, a spacecraft houses two telescopes, test masses and lasers. Other possible sources include extreme mass ratio inspirals, compact binaries, other sources form the early universe, and other theoretical objects such as cosmic strings. In 2016, LISA Pathfinder was a mission launched to test the feasibility of LISA technology. The results of this were overwhelmingly in favor of launching LISA.
LISA will be particularly sensitive to massive coalescing binaries, from hundreds to millions of times the mass of our sun. These black holes occur naturally in the centers of galaxies; merging pairs form whenever a pair of galaxies merges; and thus these LISA measurements will provide clues into how galaxies and their central black holes grow from the smallest seeds over cosmic time. Other possible sources include extreme mass ratio inspirals, compact binaries, other sources form the early universe, and other theoretical objects such as cosmic strings.
A number of CCRG members have signed onto the LISA consortium, and are dedicated to the future of gravitational wave astronomy.
Pulsar Timing Arrays
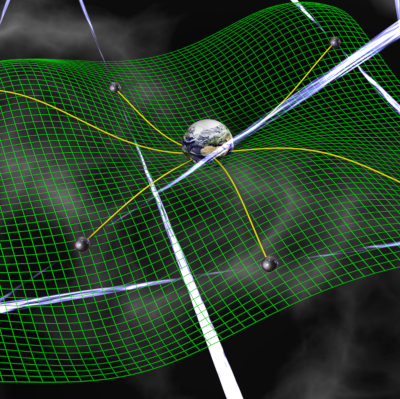
Pulsars are a class of neutron stars that spin very quickly and have a very strong magnetic field. They are still very useful to astronomy. As a result of the strong magnetic field, a jet is created and the jets emit radio waves that can be detected with ground based detectors, specifically radio telescopes. Pulsars get their name from the very periodic radio waves they emit. Therefore, if a specific pulsar or group of pulsars are off by a tiny amount, that signal can indicate that a gravitational wave passed through the pulsars on its way to Earth. Since 2017, CCRG has been performing research on pulsar timing as a means to detect gravitational waves from the inspiral of supermassive binary black holes. Those may arise as after the collision of galaxies in the Universe. Professor Lousto is a member of NANOGrav (and has been working with the Argentine Institute of Radioastronomy (IAR) to upgrade their facilities (two 30 meter antennas) to observe millisecond pulsars. Previously, the pulsar J0437-4715 was the primary focus. J0437-4715 is monitored daily and its data is reduced and analyzed by researchers at IAR. Currently, focus has shifted to J0835-4510, or Vela's pulsar. The pulsar experiences events known as glitches. Using machine learning, the pulses that emanate from this pulsar are analyzed to gain insight to the magnetosphere. Additional research in this area involves following pulsars for glitches, observation of magnetars, fast radio burst, and transient phenomena in the southern hemisphere. CCRG has a current Memorandum of Collaboration with IAR that involves exchange visits and installation of hardware in Argentina.