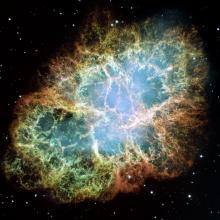
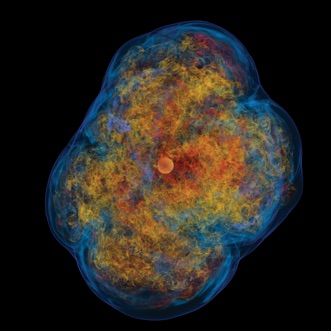
At CCRG, we are interested in studying the final fate of the inner cores of stars. Stellar cores also known as protostars form from the gravitational collapse of dense molecular clouds. The protostars rotational speed increases causing an increase in temperature and a protoplanetary disk to form around it. Later on, hydrogen is converted to helium inside the core, and then a main sequence star is formed. Once the core hydrogen is depleted, the core shrinks, and the internal temperature increases and causes the outer shell of hydrogen to ignite. The helium core contracts and increases in temperature. The star then becomes a red giant, until the core is hot enough to turn helium into carbon. What happens next depends on the mass of the star. If the star is lower mass, a white dwarf will form. If it is massive, a supernova may occur and leave behind a neutron star or just collapse into a black hole if it is even more massive.
Few events match the grandeur of supernovae and none surpass their raw power. Viewed on a cosmic scale, supernovae light up galaxies in spectacular explosions that mix the interstellar and intergalactic media. They make most of the elements in the universe, including those that form our own planet and bodies, and they give birth to the most exotic states of matter known - neutron stars and black holes. However, though supernovae have been at the forefront of astronomical research for the better part of a century, the mechanism by which they explode is not known. Supernovae are inherently multi-dimensional objects in which convection, hydrodynamic instabilities, and neutrino transport play central roles. The image on the is from a 3D core-collapse supernova simulation.
Computational work at CCRG in this area focuses on both the modeling both isolated and binary star systems which requires the use of very sophisticated, scalable software based on complex hydrodynamical models deployed on large multi-core machines.