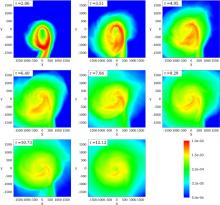
Abstract
We study how the matter dispersed when a supermassive black hole tidally disrupts a star joins an accretion flow. Combining a relativistic hydrodynamic simulation of the stellar disruption with a relativistic hydrodynamics simulation of the tidal debris motion, we track such a system until ~80% of the stellar mass bound to the black hole has settled into an accretion flow. Shocks near the stellar pericenter and also near the apocenter of the most tightly-bound debris dissipate orbital energy, but only enough to make the characteristic radius comparable to the semi-major axis of the most-bound material, not the tidal radius as previously thought. The outer shocks are caused by post-Newtonian effects, both on the stellar orbit during its disruption and on the tidal forces. Accumulation of mass into the accretion flow is non-monotonic and slow, requiring ~3--10x the orbital period of the most tightly-bound tidal streams, while the inflow time for most of the mass may be comparable to or longer than the mass accumulation time. Deflection by shocks does, however, remove enough angular momentum and energy from some mass for it to move inward even before most of the mass is accumulated into the accretion flow. Although the accretion rate rises sharply and then decays roughly as a power-law, its maximum is ~0.1x the previous expectation, and the duration of the peak is ~5x longer than previously predicted. The geometric mean of the black hole mass and stellar mass inferred from a measured event timescale is therefore ~0.2x the value given by classical theory.